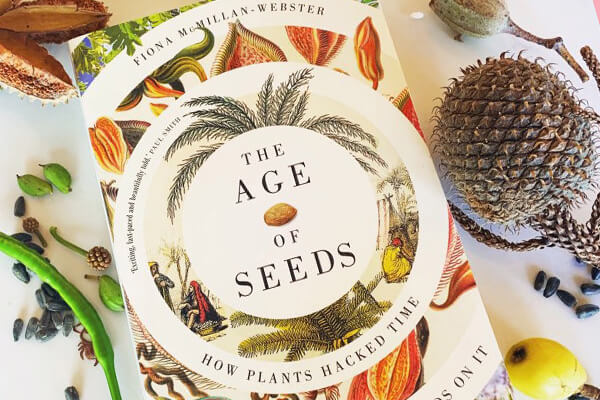
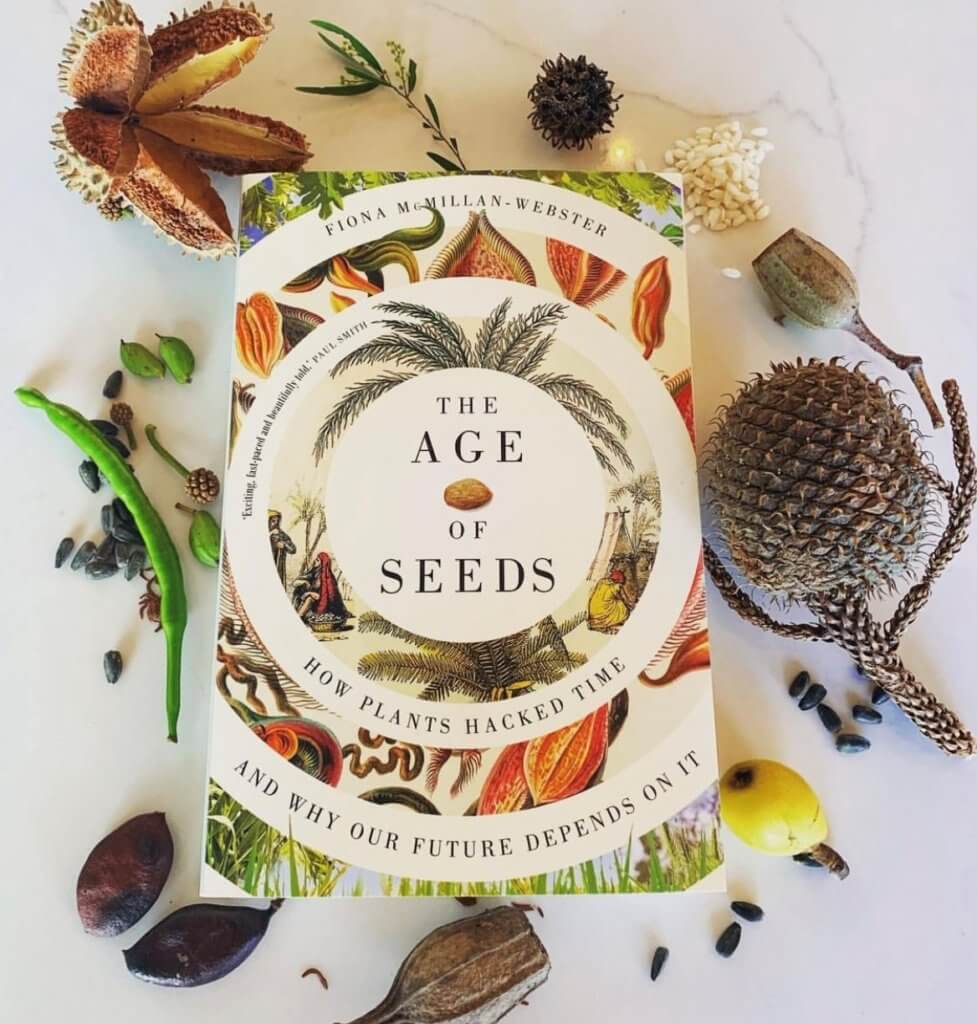
One day in 1803, although no one knows exactly which day, the human population reached one billion. It had taken our species a few hundred thousand years to arrive at that number. From there, it took just 115 years or so to double it. By 1960 we had reached three billion. Then, in just over fifty years, we hit four billion, then five billion, six billion, seven billion. That last milestone was reached on31 October 2011.
The number of people on the planet is now fast approaching eight billion, and a recent study in the British medical journal Lancet projects we’ll peak at just shy of ten billion in the year 2064. Of course, some economists wonder if the global dip in births caused by the COVID-19 pandemic might mean that the worldwide population will max out at a slightly lower figure, bringing a population inflection forward by several years. In any case, a lot more people are coming to the global dinner table, so we’re going to need a lot more food.
According to a 2018 report by the World Resources Institute, there’s a substantial shortfall between the amount of food currently produced and the amount of food we’ll need to feed all the newcomers: 7400 trillion calories to be exact. Put another way, we’re going to need to produce around 50–60 per cent more food. And the thing is, we’re not even feeding everyone adequately now. Make no mistake, we’re doing far better than we were a few decades ago. Between 1970 and 2015, undernourishment in developing countries declined from nearly 35 per cent of the population to 12.9 per cent, according to Our World in Data. And the global prevalence of hunger fell from 13.3 per cent in the year 2000 to 8.8 per cent in 2017. These are good trends, obviously. But that still leaves a lot of people who are hungry and undernourished.
It is for this reason that, in 2015, the United Nations set among its Global Goals for Sustainable Development an ambitious target of ‘Zero Hunger by 2030’. Unfortunately, a recent analysis by the UN’s own Food and Agriculture Organization (FAO) suggests this target is unlikely to be met, either by 2030 or anytime soon after. The problem, the report explains, is that although hunger incrementally decreased over decades, around 2014 this steady progress ground to a halt and since then hunger has been gradually increasing. By 2019, almost 9 per cent of the global population was undernourished, equating to around 690 million people. That was before the COVID-19 pandemic, which has only served to exacerbate food insecurity. Further analysis by the FAO has revealed that the prevalence of undernourishment reached 9.9 per cent during the first year of the pandemic, meaning that roughly one in ten people in the world were not getting adequate nutrition. The FAO projects that instead of reaching zero hunger by 2030, if current trends continue, by that time there will most likely be 840 million undernourished people in the world.
For a person facing food insecurity, it’s not just a matter of acquiring sufficient daily calories but also of getting access to adequate nutrients. Food insecurity, whether caused by crop failures or wars, has an enormous impact on diet quality. Where diets narrow to the point of undernourishment and malnourishment, health problems arise – often cruelly so. It has been estimated that over 340 million children across the world are currently deficient in micronutrients. In addition, around 22 per cent of all children under the age of five suffer stunted growth. They are not just a bit shorter than the average height for their age. Rather, stunted children are more than two standard deviations below where they should be on the World Health Organization Child Growth Standards chart. Many of these same children – around forty-five million of them – are also wasted, meaning they are profoundly underweight. The impacts on health and development are devastating. The United Nations Children’s Fund estimates that nearly half of all deaths of children under age five are due to undernourishment. Although crop failures play a role in famine, malnutrition and undernourishment, there’s certainly far more to it than that. Plenty of food is produced for human consumption every year, more than enough to feed everyone in the world. Yet one-quarter to nearly one-third of that food is wasted. This happens not only in many households but at multiple points along the global food supply chain.
To be honest, the term ‘chain’ really doesn’t provide the right visual analogy here. The global food supply is a dizzyingly complex system which is impacted at local, national and international levels by myriad trade and distribution networks, government policies, the behaviour of corporations and regulatory bodies, the mercurial prices of imports and exports, and political unrest and military conflict, as well as epidemics and, indeed, pandemics. Among these factors, conflict stands out as a particularly egregious cause of food insecurity. The Famine Early Warning Systems Network (FEWS NET), which was founded in 1985 by the United States Agency for International Development, keeps a close eye on acute food insecurity throughout the world. Currently, there are four countries on FEWS NET’s ‘highest concern’ list – Yemen, South Sudan, Nigeria and Ethiopia – comprising millions of people requiring humanitarian food assistance. Each of these crises largely has been either caused or exacerbated by protracted conflicts, where fighting – sometimes inadvertently, sometimes deliberately – has disrupted food distribution or made it difficult to plant and harvest crops in the first place. Extreme weather events and other climate-related factors often serve to make matters worse, with drought in some areas or too much rain in others causing delays in planting or crop losses. When this happens, it becomes difficult for farmers to provide even small amounts of hunger relief within the greater context of war.
This brings us to the figurative and literal root of the global food supply: plants. Their diversity and availability has enormous knock-on effects that are felt throughout the world. They provide most of the food that the world consumes, trades and fights over. Moreover, plants come with some very specific conditions for growth and survival. To get an idea of the future of food security, it’s essential to understand just how much the global food supply depends on a precariously narrow range of plants.
There are around 435,000 land plant species in the world, but humans can’t eat most of them. A few years ago, scientists at the Royal Botanic Gardens, Kew embarked on a study to better understand the scope and scale of edible plants and found that there are just 7039 plant species that are fit for human consumption. There are a few mosses and lichen on the list, but the overwhelming majority are vascular plants, most of which are seed-bearing. Altogether, these edible plants come from 272 plant families, but the spread isn’t even, with many edible plants concentrated in a small number of plant families, such as palms and grasses. Cereals, after all, are grasses. We get cocoa, okra and many other foods from the mallow family. There’s also the mustard family, which includes broccoli, cauliflower and, of course, mustard. The daisy family gives us 251 edible plants including lettuces, sunflower seeds and artichokes. Top prize goes to the pea family, which presents us with around 625 edible species.
Of course, what humans can eat and what we actually do eat are two very different things. According to the aforementioned researchers at Kew, of those 7039 edible plants, only 417 are food crops – that’s just 5.9 per cent. Furthermore, only around 250 plant species have been fully domesticated since agriculture became all the rage back in the Neolithic. Today, 90 per cent of humanity’s caloric intake comes from only fifteen crop plants, with just three – wheat, rice and maize – accounting for around half of our calories globally. As far as bottlenecks go, that’s a doozy.
We can see this reflected in seed production. Some projections suggest the global crop seed market could reach more than US$80 billion by 2025 and will continue to grow with demand. In line with our global caloric dependency, wheat, rice and maize account for around half of global seed production. Seed production plays a critical role in the global food supply. After all, most crops rely on seeds for propagation. Seed supply is itself complex and extensive, full of trade networks and a bewildering level of international distribution. In a recent statement from the International Seed Federation, an industry organisation of seed producers, its secretary-general, Michael Keller, explained, ‘Today there is no country that could fully supply farmers with seeds of their choice solely from their own production.’ In other words, every crop-growing country depends to some extent on seeds from somewhere else. And so, at any point in time, including as you’re reading this right now, shipments of seeds are crossing borders, oceans and continents. It is, effectively, human-mediated seed dispersal on a global scale. Producing such large amounts of seeds across many different parts of the world mitigates the risk of crop failures in any one location, but it still entails a reliance on a mere handful of plant species, and this makes us extremely vulnerable to food insecurity, especially in the face of a changing climate.
So, how did we get here?
Beginning around 12,000 years ago, humans narrowed their dietary diversity, gradually shifting from numerous wild, edible plants to a dependence on a much smaller number of domesticated species, and much of this took place during the relatively stable climate of the Neolithic. Nevertheless, even among that narrow cache of domesticated plants, there was still a reasonable amount of genetic diversity thanks to the many and varied locally adapted species.
For farmers, this came with benefits, explains Charlotte Lusty, who is the head of programs and genebank platform coordinator at Crop Trust, an international organisation that aims to preserve crop biodiversity. ‘If it was too hot, some varieties would die. If there was a fungus, only some of the individuals would be affected,’ she tells me. So, too, with drought, frost or flooding, says Lusty. ‘There would always be an individual there who would be resistant.’ Farmers recognised that diversity brought resilience, she adds. ‘They didn’t want uniformity because it was risky.’
Of course, the plants didn’t all flower and fruit at the same time, which is a problem if you want to harvest everything all at once. If that was the aim, then planting a field with a monoculture of a more bountiful variety of wheat, for example, provided some advantages, enabling both high yield and mass production – undeniably useful for feeding a lot of people at once as populations began to rise. There appeared to be a trade-off: you could mitigate the risk of crop failure with higher plant diversity and make do with lower yields, or you could reduce plant diversity for a shot at bigger harvests and wear the higher risk that came with that. Neither was ideal for a burgeoning global population, and by the middle of the 20th century it seemed agriculture would soon reach a breaking point.
Back in the 1950s, when the global population was fast approaching three billion, there were many grim projections about the capacity of agriculture to keep up with the demand for food. Those concerns were well founded. Even before the start of World War II, the global population was veering ever upwards, but it was after the war that birth rates really took off. By 1950, in addition to the famed post war ‘baby boom’ in places like the United States, the United Kingdom and Australia, the world’s most populous countries – China and India – began experiencing an exponential population boom that would ultimately see the addition of billions more humans before the turn of the millennium. But the food supply wasn’t keeping pace. By the early 1960s, global cereal crop production was around 750 million tonnes per annum, which was not enough to feed the coming influx. At the time, it seemed there was no way to avert a global famine. Bleak predictions warned that ‘hundreds of millions’ would starve within the space of a generation. Yet, this never happened. What changed? Well, weirdly enough, our crops got shorter.
Recall that plants spend a lot of time divvying up and redirecting resources – nutrients, carbohydrates, water. Reproduction, especially seed growth, is resource-intensive, so when the time comes, the plant directs much of its resources that way. But it can only sacrifice so much because the rest of the plant still needs energy to grow and live. Plants, of course, have many different traits, and the maintenance of those traits require energy input: maybe it’s a thorny defence mechanism or a particularly showy flower, or it might be the upkeep of a hard, woody trunk or the endless molecular negotiations between root cells and soil microbes. For plants that grow tall, there’s a lot of quiet work involved in stem growth, including all the plumbing and structural support that ensures the plant doesn’t topple under its own weight. For long-stemmed cereal grasses, like wheat, rice and maize, it’s not good to be both tall and top-heavy. Yet, the top is where the grains grow, all those endosperm-filled seeds that sustain us. And so it was that humans had cultivated cereal plants that grew tall but which, in being tall, had limits on the quantity of grains they could produce without the plant buckling under its own weight. This problem, which plant breeders and farmers call ‘lodging’, makes harvesting very difficult and leads to substantial crop waste. So, even though the first half of the 20th century had seen the development of industrial processes that enabled large-scale fertiliser production, just throwing a whole bunch of fertiliser on the world’s cereal crops wasn’t helping as much as hoped. It turned out that high-fertiliser regimes cause cereals to grow too quickly, leading to weak stems, which made the lodging problem worse. In a sense, the real problem, and the source of all those fears of global famine, was gravity.
The solution began to reveal itself in Japan in 1935 when a plant breeder by the name of Gonjiro Inazuka took a short-strawed native Japanese wheat called Daruma and crossbred it with high-yielding varieties of American wheat. The result was a new wheat variety called Norin-10. Not long after World War II, Orville Vogel, a plant breeder at Washington State University, acquired samples ofNorin-10 and used them to develop a high-yielding dwarf variety of winter wheat. This was good progress, but it wasn’t of much use to farmers in warmer climates. Then, in the early 1950s, some of Vogel’s new lines were sent to Norman Borlaug, who was breeding rust-resistant wheat at what would later become the International Maize and Wheat Improvement Center in Mexico. Intrigued by Vogel’s new wheat variety, Borlaug began crossing it with Mexican varieties that grew well in the local climate. His work led to the first high-yielding semi-dwarf wheat that grew well in tropical and subtropical climates. It had short, strong stems and produced large numbers of tillers, which are the protrusions on which the wheat heads grow. It not only produced more wheat heads, but each head also produced more individual grains than before.
Though neither Inazuka, Vogel or Borlaug knew it at the time, the success of this venture hinged on the presence of Rht genes which had been passed down from the Japanese Daruma wheat. It’s now known these genes reduce a growing plant’s response to gibberellins, those very same hormones involved in seed development and germination. The presence of Rht genes resulted in wheat with shorter, stronger stalks yet far more grains. Importantly, this wheat didn’t ‘lodge’. Moreover, while Borlaug’s new wheat variety also grew in warm climates, because it did not require exposure to cold temperatures in order to flower and produce grains, farmers could produce two crops each year instead of one. Borlaug shared his wheat with countries in Central America, South America and South-East Asia and, as a result, crop yields went through the proverbial roof. In Mexico alone, grain production doubled within seven years.
Meanwhile, a similar story was unfolding in rice breeding. In the1960s, production of domesticated O. sativa was averaging around 4 tonnes per hectare, but this was not keeping pace with demand. Again, the key to success lay in interfering with gibberellins. In rice, it all started with a Chinese variety of dwarf indica rice, Dee-geo-woo-gen. It turns out that Dee-geo-woo-gen possessed a mutation in a gene called sd1. Normally, sd1 plays an important role in the production of gibberellins in plant cells. Mutations in sd1 can throw a spanner in the works and the plants don’t produce enough gibberellins to grow tall. Dee-geo-woo-gen was used by plant breeders to create shorter, stronger, yet high-yielding varieties of rice, and these went on to be the basis of numerous commercial varieties grown in the tropics and subtropics, as well as in temperate areas.
Another advancement came not long after the first semi-dwarf rice varieties were bred. Chinese agricultural scientist Yuan Longping discovered that heterosis, or hybrid vigour, was possible in rice. It’s a phenomenon in which the offspring of two very different plant parents are much more robust and productive than either parent. This had been researched and exploited in other crops, such as corn, but it was not thought possible in rice until Longping’s discovery. Achieving heterosis was tricky at a large scale because, unlike those other crops, rice is self-pollinating. The tiny flowers have the male and female parts so close together that it’s essentially impossible to pollinate an entire field of rice plants with pollen from another variety. But Longping was determined – he had seen first-hand people dying of starvation during the Great Chinese Famine in the early 1960s, and he wanted to make sure nothing like that ever happened again. He searched for years for a rare mutant that would make cross-pollination possible, and in 1970 he finally found one on an island off China’s southern coast, in a strain of wild rice. A mutation had rendered it unable to make its own pollen, but the female reproductive structures were working just fine. Without that rice’s own pollen to get in the way, large-scale hybridisation suddenly became a lot more feasible. Longping and his colleagues used this as the basis of a breeding system that led to the extensive production of new, high-yield, hybrid rice varieties that displayed hybrid vigour. The result was a 20 per cent increase in annual rice production, which has had an enormous impact on global food security.
The arrival of high-yielding dwarf cereals in the 1960s, 1970s and 1980s ushered in a world of cereal crops that not only produced more grains but were also structurally more amenable to mechanised harvesting, making large-scale cultivation easier. This era in agriculture has been called the Green Revolution because it resulted in global food production that kept up with, and even exceeded, the demands of a swelling population of humans, as well as a growing population of livestock used for dairy and meat production. It irrevocably changed farming, and the surface of the planet along with it.
This is an extract from The Age of Seeds by Fiona McMillan-Webster
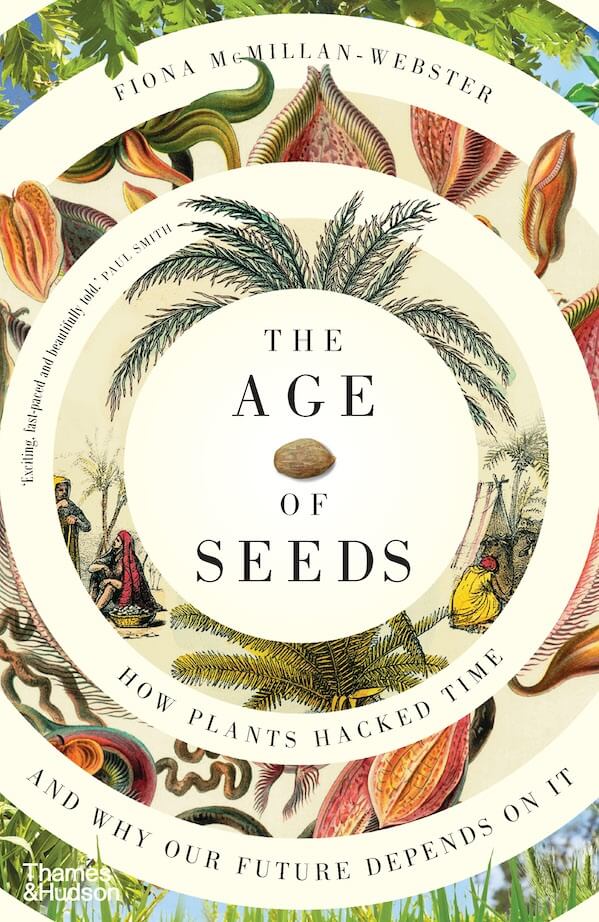
The Age of Seeds by Fiona McMillan-Webster is available now.
AU $34.99
Posted on July 24, 2022